Galactose is a six-carbon monosaccharide.
Isolated for the first time by Louis Pasteur in 1855 from milk, its structure was clarified thirty years later by Morrell R. and Fischer E.[15] In 1860, Berthelot P.E.M. named it as lactic glucose or galactose, from the Greek galaktos, which means milk, followed by the suffix for sugars –ose.[2]
It occurs in both open-chain and cyclic form. The open chain form has a carbonyl group at one end of the chain, which makes it an aldehyde derivative, therefore an aldohexose and a reducing sugar. In the cyclic form, it has four stereoisomers.[14]
The main dietary source is the disaccharide lactose, which is made up of a galactose molecule and a glucose molecule joined by a β-(1→4) glycosidic bond. In the small intestine, the glycosidic bond is hydrolyzed in a reaction catalyzed by lactase (EC 3.2.1.108) to produce galactose and glucose, which are then absorbed.[18]
It performs a wide range of functions. After conversion to UDP-glucose and UDP-galactose, through the Leloir pathway,[11] it can be used for both anabolic and catabolic purposes, depending on the type of tissue and the energy state of the cell.[5]
A defect in one of the enzymes of the Leloir pathway leads to the abnormal accumulation of the galactose-related chemicals galactitol and galactonate in many tissues and organs, and causes galactosemia.[22]
Contents
- Chemical properties
- Food sources
- Intestinal absorption
- Metabolism of galactose
- Galactosemia
- References
Chemical properties
Like all hexoses, such as glucose and fructose, its molecular formula is C6H12O6 and molecular weight 180.156 g/mol.
It is about 33 percent as sweet as sucrose, and about half as sweet as glucose.[13]
Like all aldohexoses, galactose has two enantiomers that, based on Fischer-Rosanoff convention or D-L system, are called D-galactose and L-galactose. The latter is not naturally occurring in higher organisms as it cannot be further metabolized. Therefore, the term galactose will be used to indicate D-galactose.
It can occur in linear or open-chain form and in cyclic form. The open chain form, which may be represented by a Fischer projection, has a aldehyde group, hence a carbonyl group, at one end of the chain.
The carbonyl group makes it a reducing sugar, like the other monosaccharides and the disaccharides lactose and maltose.[14]
Structure
The open-chain form is thermodynamically unstable and is present in trace amounts in solution.
Aldehydes can react with alcohols, so the linear form of galactose can cyclize via nucleophilic attack by the oxygen atom of one of the hydroxyl groups to the carbon atom of the carbonyl group to form an intramolecular hemiacetal.[19]
Cyclic compounds with rings consisting of three or four atoms are much less stable than the those consisting of five and, above all, six atoms, due to the high steric interactions, like the cyclic alkanes cyclopropane and cyclobutane which are less stable than cyclopentane and cyclohexane.
In the cyclic form, galactose exists as four stereoisomers.[20] Two have a five-member ring consisting of one oxygen atom and four carbon atoms, namely, a furanose ring, and two have a six-member ring consisting of one oxygen atom and five carbon atoms, namely, a pyranose ring.
In solution, pyranose and furanose forms are in equilibrium with the open chain form but, since the furanose form is less stable than the pyranose form, the equilibrium is strongly shifted towards the latter.[14]
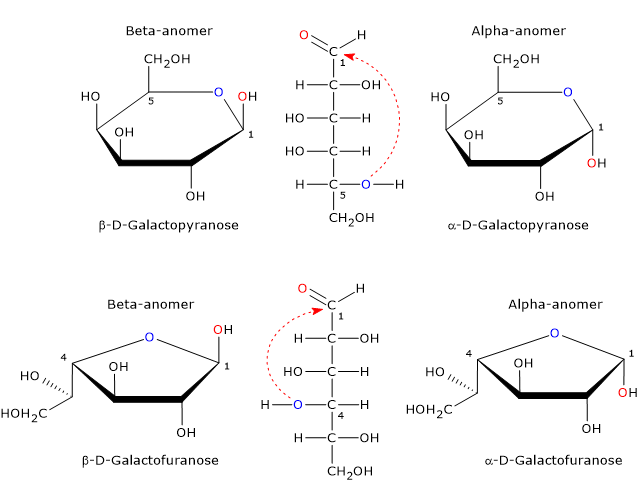
The only cyclic form found in mammals is the pyranose form, which is a epimer of glucose as it differs only by the configuration of the hydroxyl group on the C4. The furanose form is found in many lower organisms, both prokaryotes and eukaryotes, such as bacteria, green algae, fungi, protozoa, such as Trypanosomatids, sponges and starfish. Furanose form is also found in pathogenic species where it may play an important role, for example for survival, while its biosynthetic pathway may be a target for the development of new drugs to treat infections caused by these pathogens.[20]
Anomers
Hemiacetal carbon, a tetrahedral atom that bear four different ligands, is a center of chirality.
Since nucleophilic attack by the oxygen atom can occur from topside or bottom site of the sp2 plane of the carbonyl carbon, the ring-closing reaction may yield two pyranose configurations and two furanose configurations which differ in the configuration of the hemiacetal carbon. This phenomenon is called mutarotation and yields two pair of epimers.
Such epimers differ only in the configuration of the hemiacetal carbon and are called alpha-anomer and beta-anomer, while the hemiacetal carbon becomes the anomeric carbon or anomeric center.
Alpha-anomer is formed via the nucleophilic attack from topside of the sp2 plane, whereas beta-anomer via the nucleophilic attack from bottom side of the sp2 plane.
Therefore, galactose can occur in five different forms: the open chain form plus four anomeric cyclic forms, that is, α-D-galactopyranose and β-D-galactopyranose, and α-D-galactofuranose and β-D-galactofuranose.[19]
Note: anomerism is a type of optical isomerism characteristic of monosaccharides.
Food sources
The main dietary sources are milk and dairy products, where it is mainly found as a component of lactose, and to a less extent, of more complex carbohydrates, such as oligosaccharides and polysaccharides, called glycans, components of milk glycoproteins and glycolipids.[7]
It is also found in many other foods, such as fruit, vegetables, cereals, legumes, nuts and honey, in which it can occur in free form, bound to polyphenols, such as flavonols and anthocyanins, or as a components of glycoproteins and glycolipids.[6] In the bound form, it is often linked by chemical bonds resistant to digestion.[1]
In the free form, it occurs in milligram amounts; below some examples:
- less than 0.1 mg/100 g of edible portion: artichokes, mushrooms, olives, and peanuts;
- more than 10 mg/100 g of edible portion: peppers, date, papaya, watermelon, tomato;
- up to 35.4 mg/100 g of edible portion in persimmon.
Although these values are very low, they must be considered in case of galactosemia.[8]
Intestinal absorption
In mammals, carbohydrate digestion begins in the mouth and ends in the duodenum and jejunum, where hydrolases, such as pancreatic alpha-amylase and the disaccharidases of the brush border of enterocytes, hydrolyze disaccharides, oligosaccharides and polysaccharides into the constituent monosaccharides: glucose, fructose and galactose.[18]
The hydrolysis of lactose to produce glucose and beta-galactose is catalyzed by the disaccharidase lactase or lactase phlorizin-hydrolase. The enzyme has two active sites: one catalyzes the hydrolysis of the β-(1→4) glycosidic bond, therefore it is a beta-glucosidase, and one catalyzes the hydrolysis of phlorizin and glycolipids, such as ceramides to yield fatty acids and sphingosine.[24] Bacterial beta-galactosidase (EC 3.2.1.23) can hydrolyze lactose into the two monosaccharides, too.[17]
The absorption of monosaccharides glucose and galactose takes place in the small intestine, where it occurs through the sodium-glucose linked transporter type I or SLGT1, an integral membrane protein which mediates their active transport across the brush border membrane of enterocytes. The transmembrane electrochemical gradient of sodium ions is the energy source for this transport system.[23]
Galactose leaves the enterocyte through the basolateral membrane by facilitated diffusion, mediated by the glucose transporter type 2 or GLUT2, enters blood circulation and is carried through the portal system to the liver, that absorbs approximately 90 percent of the circulating monosaccharide The uptake into hepatocytes is mediated by GLUT2.[23] Galactose not absorbed by the liver can reach other organs, such as the lactating mammary gland.
Under physiological conditions, blood galactose concentration may increase following alcohol intake, which reduces both its intestinal absorption and its hepatic metabolism.
Metabolism of galactose
In the absence of lactose or other dietary sources of galactose, the monosaccharide can be synthesized from glucose, as UDP-galactose 4-epimerase (EC 5.1.3.2), one of the four enzymes of the Leloir pathway, catalyzes a reversible reaction.[18]
In addition to the de novo synthesis and dietary intake, galactose deriving from the turnover of glycoproteins and glycolipids is very important.[4]
Galactose may enter three main metabolic pathways, the most important of which is the Leloir pathway, by which it may be converted to glucose. The other two pathways, an oxidative one that leads to the formation of galactonate, and a reductive one that leads to the conversion of galactose into galactitol, play marginal roles under physiological conditions, whereas they may be substantially fueled in the presence of excessive levels of galactose, as in galactosemia.[5]
Leloir pathway
The Leloir pathway, discovered by Leloir L.F. and colleagues in 1948, through four enzymatic reactions leads to the inversion of the configuration of hydroxyl group at C4 of galactose to give glucose.[11]
- Beta galactose, a product of lactose digestion, is converted to the alpha anomer in a reaction catalyzed by galactose mutarotase (EC 5.1.3.3).[21]
- In the next step, galactokinase (EC 2.7.1.6) catalyzes the phosphorylation of galactose to galactose-1-phosphate, which cannot diffuse out of the cell.[9] Furthermore, galactose phosphorylation maintains a low intracellular concentration of the monosaccharide favoring its transport into the cell.
- Galactose-1-phosphate is activated to UDP-galactose in a reaction catalyzed by galactose-1-phosphate uridylyltransferase (EC 2.7.7.12). Finally, UDP-galactose is epimerized to UDP-glucose in a reaction catalyzed by UDP-galactose 4-epimerase.[9]
Leloir pathway allows the activation of galactose to UDP-galactose that may be used as sugar donor for glycosylation reactions of lipids and proteins.[5] This allows galactose to plays a key structural role in the early stages of development, and to be a component of antigens present on erythrocytes, in particular those used to determine the AB0 group. In lactating mammary gland, UDP-galactose is a precursor for lactose synthesis, accounting for about 30% of the galactose in lactose, and is used in glycosylation reactions of milk lipids and proteins.[18]
UDP-glucose, depending on the type of tissue and the energy state of the cell, may be used in glycosylation reactions, in glycogen synthesis, which is the main metabolic fate of galactose in the liver, it may enter glycolysis to be used for energy or in gluconeogenesis, after conversion to glucose-1-phosphate in a reaction catalyzed by UDP-glucose pyrophosphorylase (EC 2.7.7.9) and subsequent isomerization to glucose-6-phosphate in a reaction catalyzed by phosphoglucomutase (EC 5.4. 2.2).[4][5]
As UDP-glucose pyrophosphorylase catalyzes a reversible reaction, the synthesis of UDP-galactose from galactose-1-phosphate and UTP may be an alternative metabolic pathway in subjects with deficiency of galactose-1-phosphate uridylyltransferase.[12]
Galactitol
An alternative metabolic pathway to the Leloir pathway leads to the reduction of galactose to galactitol, in a reaction catalyzed by aldose reductase (EC 1.1.1.21), a NADPH dependent reductase with broad substrate specificity toward various monosaccharides, being also able to reduce glucose to sorbitol.[4]
Aldose reductase has a low affinity for galactose, so that under physiological conditions, galactitol is present in traces, whereas, in case of abnormal galactose accumulation, as in galactosemia, its production increases.
Sorbitol can be converted to fructose in a reaction catalyzed by sorbitol dehydrogenase (EC 1.1.99.21), whereas galactitol is not further metabolized and accumulates in the cell as it is poorly fat soluble molecule, therefore unable to cross the plasma membrane.[5]
Note: the metabolic pathway that leads to the conversion of glucose to fructose is called the polyol pathway.
Galactonate
Another alternative route to the Leloir pathway leads to the oxidation of galactose to galactonate.[4]
- In the first step of the pathway, galactose is oxidized to galactone-1,4-lactone, in a reaction catalyzed by galactose dehydrogenase (EC 1.1.1.48); in the reaction NAD+ acts as an electron acceptor and is reduced.
- In the second step, the lactone is hydrolyzed to galactonate, in a reaction which can proceed spontaneously or be catalyzed by a lactonase (EC 3.1.1.25). Galactonate can be excreted in the urine or further oxidized to β-keto-galactonate in a reaction catalyzed by β-L-hydroxy acid dehydrogenase. The reaction leads to further production of NADH.
- In the last step, β-keto-galactonate is decarboxylated to xylulose, in a reaction catalyzed by a decarboxylase not yet been identified, with release of the C1 carbon as CO2.
Finally, xylulose may enter the pentose phosphate pathway following phosphorylation to xylulose-5-phosphate in a reaction catalyzed by ATP:D-xylulose-5-phosphotransferase or xylulokinase (EC 2.7.1.17).[10]
Galactosemia CIT OK
Galactosemia is a genetic metabolic disorder caused by a defect in one of the genes encoding the enzymes of the Leloir pathway.
First described by von Reuss A. in 1908, it is characterized by an abnormal accumulation of galactonate and galactitol in many organs of the body.[22]
The symptoms include cataracts, which often appear early, within the first two years of life, while, in the most severe cases, kidneys, liver and brain are also damaged.
Regarding cataracts, the synthesis and accumulation of galactitol in the lens seems to be one of the factors that triggers the disease, as:
- being osmotically active, it causes an increase in osmotic pressure and then a net flow of water inside the cell, which may lead to osmotic lysis of the cytoplasmic membrane;[4]
- its synthesis depletes NADPH levels that, by reducing the activity of glutathione reductase (EC 1.8.1.7), leads to free radical accumulation and oxidative stress, which may cause cell death.[16]
To date, the therapeutic standard of care for galactosemia is a galactose-restricted diet.[3]
References
- ^ Acosta P.B., Gross K.C. Hidden sources of galactose in the environment. Eur J Pediatr 1995;154(7 Suppl 2):S87-92. doi:10.1007/BF02143811
- ^ Berthelot M. Chimie organique fondée sur la synthèese Vol. 1, 1860. Mallet-Bachelier
- ^ Coelho A.I., Berry G.T., Rubio-Gozalbo M.E. Galactose metabolism and health. Curr Opin Clin Nutr Metab Care 2015;18(4):422-427. doi:10.1097/MCO.0000000000000189
- ^ a b c d e Coelho A.I., Rubio-Gozalbo M.E., Vicente J.B., Rivera I. Sweet and sour: an update on classic galactosemia. J Inherit Metab Dis 2017;40(3):325-342. doi:10.1007/s10545-017-0029-3
- ^ a b c d e Conte F., van Buuringen N., Voermans N.C., Lefeber D.J. Galactose in human metabolism, glycosylation and congenital metabolic diseases: time for a closer look. Biochim Biophys Acta Gen Subj 2021;1865(8):129898. doi:10.1016/j.bbagen.2021.129898
- ^ de la Rosa L.A., Alvarez-Parrilla E., Gonzàlez-Aguilar G.A. Fruit and vegetable phytochemicals: chemistry, nutritional value, and stability. 1th Edition. Wiley J. & Sons, Inc., Publication, 2010
- ^ Flynn A. Galactosemia. Editor(s): McSweeney P.L.H., McNamara J.P. Encyclopedia of dairy sciences. 3th Edition. Academic Press, 2022;853-858. doi:10.1016/B978-0-12-818766-1.00094-5
- ^ Gross K. C., Acosta P. B. Fruits and vegetables are a source of galactose: implications in planning the diets of patients with galactosaemia. J Inherit Metab Dis 1991;14(2):253-258. doi:10.1007/BF01800599
- ^ a b Holden H.M., Rayment I., Thoden J.B. Structure and function of enzymes of the Leloir pathway for galactose metabolism. J Biol Chem 2003;278(45):43885-43888. doi:10.1074/jbc.r300025200
- ^ Lai K., Klapa M.I. Alternative pathways of galactose assimilation: could inverse metabolic engineering provide an alternative to galactosemic patients? Metab Eng. 2004 Jul;6(3):239-44. doi:10.1016/j.ymben.2004.01.001
- ^ a b Leloir L.F., de Fekete M.A., Cardini C.E. Starch and oligosaccharide synthesis from uridine diphosphate glucose. J Biol Chem 1961;236:636-41. doi:10.1016/S0021-9258(18)64280-2
- ^ Leslie N., Yager C., Reynolds R., Segal S. UDP-galactose pyrophosphorylase in mice with galactose-1-phosphate uridyltransferase deficiency. Mol Genet Metab 2005;85(1):21-7. doi:10.1016/j.ymgme.2005.01.004
- ^ National Center for Biotechnology Information. PubChem Compound Summary for CID 6036, D-Galactose. https://pubchem.ncbi.nlm.nih.gov/compound/D-Galactose. Accessed Dec. 10, 2023
- ^ a b c Nelson D.L., Cox M.M. Lehninger. Principles of biochemistry. 6th Edition. W.H. Freeman and Company, 2012
- ^ Pasteur L. Note sur le sucre de lait. 1856
- ^ Pintor J. Sugars, the crystalline lens and the development of cataracts. Biochem Pharmacol 2012;1:4. doi: 10.4172/2167-0501.1000e119
- ^ Richmond M.L., Gray J.I., and Stine C.M. Beta-galactosidase: review of recent research related to technological application, nutritional concerns, and immobilization. J Dairy Sci 1981;64:1759-1771. doi: 10.3168/jds.S0022-0302(81)82764-6
- ^ a b c d Rosenthal M.D., Glew R.H. Medical biochemistry: human metabolism in health and disease. A John Wiley & sons, Inc., Publication, 2009
- ^ a b Soderberg T. Organic chemistry with a biological emphasis. Volume II. Chemistry Publications. 2019 https://digitalcommons.morris.umn.edu/chem_facpubs/2
- ^ a b Tefsen B., Ram A.F.J., van Die I., Routier F.H. Galactofuranose in eukaryotes: aspects of biosynthesis and functional impact. Glycobiology 2012;22(4):456-469. doi:10.1093/glycob/cwr144
- ^ Thoden J.B., Timson D.J., Reece R.J., and M. Holden H.M. Molecular structure of human galactose mutarotase. J Biol Chem 2004;279(22):23431-23437. doi:10.1074/jbc.M402347200
- ^ a b Timson D.J. Type IV galactosemia. Genet Med 2019;21:1283-1285. doi:10.1038/s41436-018-0359-z
- ^ a b Wright E.M., Hirayama B.A., Loo D.F. Active sugar transport in health and disease. J Intern Med 2007;261(1):32-43. doi: 10.1111/j.1365-2796.2006.01746.x. PMID: 17222166
- ^ Zecca L., Mesonero J.E., Stutz A., Poirée J.C., Giudicelli J., Cursio R., Gloor S.M., Semenza G. Intestinal lactase-phlorizin hydrolase (LPH): the two catalytic sites; the role of the pancreas in pro-LPH maturation. FEBS Lett 1998;435(2-3):225-8. doi: 10.1016/s0014-5793(98)01076-x