Sucrose or saccharose or alpha-D-glucopyranosyl-(1→2)-beta-D-fructofuranoside is sugar and a disaccharide made up of the monosaccharides alpha-D-glucose, in the pyranose form, and beta-D-fructose, in the furanose form. The monosaccharides are joined by α-(1→2) glycosidic bond, an acetal oxygen bridge from hemiacetal of glucose to the hemiketal of the fructose. This glycosidic bond occurs with retention of C1 configuration, hence alpha configuration.
As with lactose, maltose and trehalose, three other disaccharide, its molecular formula is C12H22O11 and molecular weight is 342.30 g/mol. It appears as white odorless crystalline solid.[7]
It is a non-reducing sugar since the α-(1→2) glycosidic bond occurs between the aldehyde function of glucose and the ketone functional group of fructose, that is, their anomeric carbons. This means that, in solution, it cannot exist in an open chain form, hence, there are no free carbonyl groups. Trehalose is a non-reducing sugar, too.
It is produced by algae, cyanobacteria and plants, and is the end product of photosynthesis, the main metabolic pathway for fixing carbon on Earth.[5][11] Once transported through the phloem to non-photosynthetic tissues, it can be used by plants as an energy source as well as a precursor for the synthesis of several compounds including amino acids, and therefore proteins, cellulose, starch, and nucleotides. Furthermore, it is involved in the regulation of gene expression.[10]
It occurs in practically all plants, but its extraction is economically advantageous only from sugar beets (Beta vulgaris ssp. vulgaris var. altissima) and sugarcane (Saccharum officinarum). Except for the initial steps, the extraction is very similar from both sources.[12][13]
Despite competition from artificial sweeteners, sucrose is still the most widely used sweetener for both domestic and industrial use. Moreover, thanks to its antimicrobial action, which is exerted at high concentrations, it is one of the most used preservatives, for example for jams and marmalades.
With starch and lactose, it is one of the three most common dietary carbohydrates.
Contents
- History
- Biosynthesis
- Role in plants
- Food sources
- Extraction from sugarcane
- Extraction from sugar beet
- Intestinal digestion
- Sucrase-isomaltase deficiency
- References
History
Sucrose seems to have been discovered in India in the 6th century BC, as a result of the boiling of juice from the sugarcane. The army of Alexander the Great, among Westerners, was the first to come into contact with it. However, it remained a rare commodity for over a millennium, and it was after the Crusades that it gained importance as a sweetener, albeit only among wealthier classes.[9]
In 1747 Marggraf A.S., a German chemist, discovered sucrose in sugar beet, and in 1802 Achard F., a student of his, developed industrial process for its extraction. The same process will be fitted, with minor changes, to sugarcane.
The word saccharose was coined in 1860 by Berthelot M., a French chemist, the same who isolated trehalose from Trehala manna, whereas the word sucrose was coined by the English chemist Miller W. three years earlier, in 1857.
Biosynthesis
Sucrose is the end product of photosynthesis, a process which, using light energy, water and inorganic carbon in the form of carbon dioxide, leads to the transformation of light energy into chemical energy and fixation of carbon, namely, leads to the biosynthesis of energy-rich organic compounds. In addition, photosynthesis releases molecular oxygen to the environment.[11]
In plants, carbon dioxide is fixed in chloroplast stroma through the Calvin cycle or the dark reactions of photosynthesis to yield triose phosphates. Triose phosphates can remain in the chloroplast and be converted into starch, which will then be used in the following night, or be transported to the cytosol by a specific transporter, via an antiport with phosphate ions.[5]
In the cytosol, fructose 1,6-bisphosphate aldolase or simply aldolase (EC 4.1.2.13) catalyzes the aldol condensation between two molecules of triose phosphate, dihydroxyacetone phosphate and glyceraldehydes 3-phosphate, to form fructose 1,6-bisphosphate.
Fructose 1,6-bisphosphate can be metabolized to yield other hexose phosphates such as fructose 6-phosphate, that, in turn, can be isomerized to glucose 6-phosphate, in a reversible isomerization reaction catalyzed by phosphoglucose isomerase (EC: 5.3.1.9).
Aldolase and phosphoglucose isomerase are enzyme also involved in glycolysis, in the reverse reactions, and gluconeogenesis.[8]
Glucose 6-phosphate is isomerized to glucose 1-phosphate, in a reversible reaction catalyzed by phosphoglucomutase (EC 5.4.2.2), an enzyme also involved in glycogen synthesis and glycogenolysis.
Glucose 1-phosphate can be activated by binding UTP to the anomeric carbon to form UDP-glucose, in a reaction catalyzed by UDP-glucose pyrophosphorylase (EC 2.7.7.9).
UDP-glucose is a nucleotide sugar that plays a central role in carbohydrate metabolism; for example it is involved in glycogen synthesis, galactose metabolism, after conversion in UDP-galactose, and, in photosynthetic organisms, in sucrose biosynthesis.
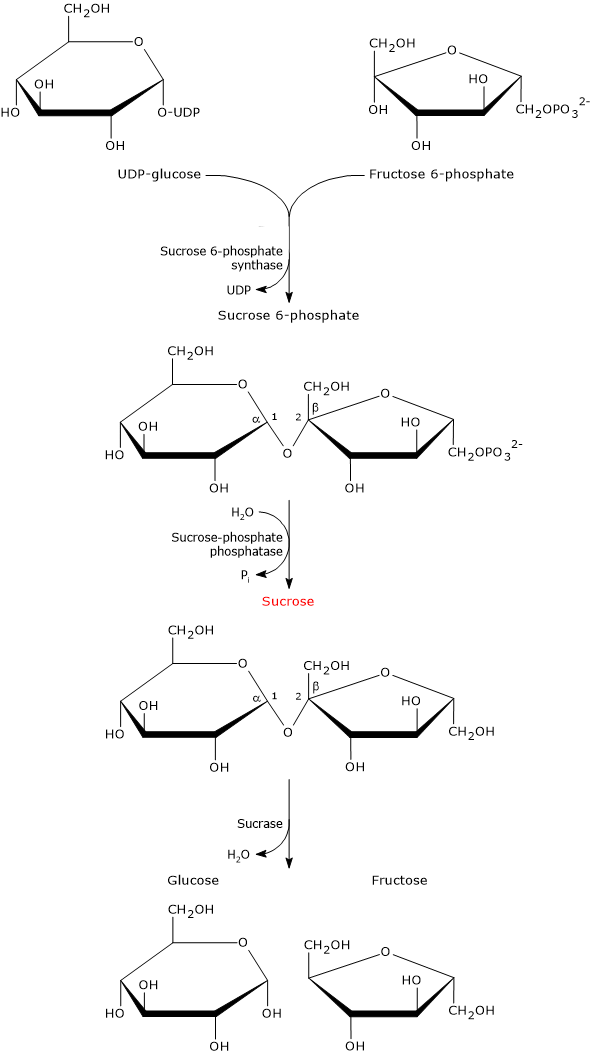
UDP-glucose and fructose 6-phosphate are the building blocks for the biosynthesis of sucrose, which occurs in two steps.
In the first step, sucrose 6-phosphate synthase (EC 2.4.1.14) catalyzes a reversible reaction in which the sugar moiety of UDP-glucose is transferred to fructose 6-phosphate to form sucrose 6-phosphate.
Sucrose 6-phosphate is dephosphorylated to sucrose in a irreversible hydrolysis catalyzed by sucrose-phosphate phosphatase (EC 3.1.3.24).[1] This hydrolysis shifts the equilibrium of the reaction catalyzed by sucrose 6-phosphate synthase towards sucrose biosynthesis.
Regulation of biosynthesis
The biosynthesis of sucrose is highly regulated and coordinated with the biosynthesis of starch, which occurs in chloroplasts.
Sucrose 6-phosphate synthase, which is also involved in starch metabolism, is the key regulatory enzyme, and is regulated via allosteric effectors and covalent modifications, that is, reversible phosphorylations. And, as with the regulation of glycolysis and gluconeogenesis, fructose 2,6-bisphosphate plays a key role.[5]
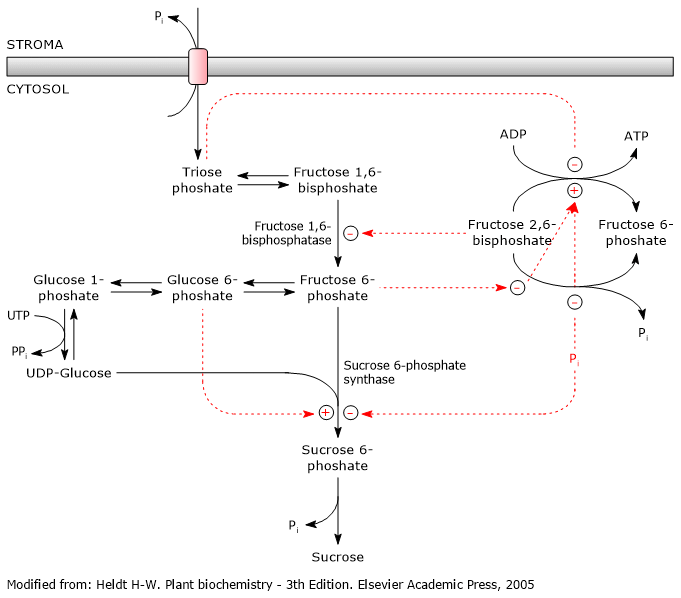
It also appears that trehalose 6-phosphate is involved, whose role would be to regulate and signal sucrose levels by acting as a negative feedback regulator.
Role in plants
Sucrose is the major product of photosynthetic tissues and is the main sugar transported through the phloem to the non-photosynthetic tissues of the plant.
In non-photosynthetic tissues, it can enter different metabolic pathways such as those leading to the production of starch, cellulose, amino acids, polyphenols, nucleotides, fatty acids, lipids, carotenoids, and many other compounds, or can be used for energy.
It is also involved in plant response to abiotic stress and in transcriptional and post-transcriptional control of gene expression.[10]
In Tracheophytes, also called vascular plants, it performs many of the functions that trehalose performs in bacteria, fungi and insects, and its concentration is 100 to 1000 times higher than that of trehalose. The reasons for this primacy are uncertain. It has been suggested that the lower viscosity of concentrated sucrose solutions may have made it more suitable than trehalose for transport in the phloem.[6]
Food sources
Sucrose, being the end product of photosynthesis, is widely spread in fruit and vegetables, together with fructose and glucose. However, these three carbohydrates are present in different amounts. For example, glucose and fructose are the major sugars in tomatoes, grapes, blueberries, blackberries, cherries, figs, avocados, and lemons, whereas sucrose is practically absent; conversely, sucrose is the major sugar in bananas, peaches, oranges, mangoes, sweet corn, sweet peas, and carrots.[2]
Among natural sweeteners, it is the major sugar in maple syrup and molasses, where it accounts for over 98 percent and about 53 percent of total sugars, respectively, whereas it constitutes about 14 percent and just over 1 percent of total sugars of agave syrup and honey.
Extraction from sugarcane
Sugarcane accounts for about 75 percent of global sucrose production, and the disaccharide makes up about 10-15 percent of the weight of plants ready to be harvested.[2]
Once cut, the plant must be rapidly processed, as it deteriorates very quickly.
In the first step of processing, sugarcane is grinded and crushed for juice extraction. The fibrous plant residue, called bagasse, once dried, can be used as a fuel.[13]
The next step is the filtration step. To avoid that sucrose is cleaved into fructose and glucose by organic acids, which would lead to the formation of invert sugar, calcium hydroxide is added.
The neutralized juice is heated up to 95° C (200° F) and clarified by calcium hydroxide, that causes a precipitation, even if incomplete, of impurities such as glucose, fructose, fiber, pectins, inorganic ashes, amino acids, proteins and other compounds, forming a mud which is separated by gravity or centrifugation. By heating, a partial water evaporation occurs, that reaches a concentration of about 35 percent of the juice. Calcium ions, derived from calcium hydroxide, are removed by bubbling carbon dioxide through the juice. The reaction between carbon dioxide and ion calcium produces a calcium carbonate precipitate.
The following step is the crystallization by which, through a series of centrifugation and evaporation steps, sucrose is separated from the molasse: the product is raw cane sugar ready for sale.
Molasses is one of the additives that can be added to animal feed; moreover, it can be used to produce ethanol, citric acid, yeast tablets, and rum.
Refined sugar
Refined sugar is produced from raw cane sugar through separation of residual molasse, which has an negligible nutritional value.
After the sugar has been dissolved hot water, calcium hydroxide is added to precipitate residual molasses and then obtain a further clarification.
At this point, the solution, which contains yellowish residues, is passed through activated carbon that absorbs the residues.
Refined sugar is eventually obtained through a series of crystallizations and centrifugations.
Extraction from sugar beet
Sucrose content of sugar beet ready to be harvested, thanks to selection efforts in the last two centuries, passed from 4.5 percent to 16-18 percent of the weight of the plant. Its cultivation is particularly important in Europe.[2][12]
Sucrose extraction from sugar beets is similar to the extraction from sugar cane.
After being harvested and washed, sugar beets are cut into slices and put in water at a temperature of from 60° to 70° C (140°-158° F). This leads to the rupture of cell membranes and the release of sucrose. The solution, called raw juice, contains, in addition to sucrose in a concentration between 10 percent and 15 percent, many impurities, both inorganic, as salts, and organic, as glutamic acid, proteins, including oxidative enzymes, polyphenols, acids, saponins, betaines, pectins, which give it a color ranging from brownish to black, and that must be removed.
The heat-resistant microorganisms are eliminated by adding a disinfectant, for example sulfur dioxide, which will be re-added in a subsequent step to prevent browning and degradation reactions.
Like for sugarcane processing:
- Calcium hydroxide is used for precipitating impurities;
- Carbon dioxide is bubbled through the juice to precipitate calcium carbonate that carries with it some of the impurities;
- evaporation and crystallization steps yield to the production of a sugar with a purity higher than 99.7 percent.
Intestinal digestion
In mammals, carbohydrate digestion takes place mainly in the duodenum, the portion of the intestine downstream the stomach.
Hydrolases of the brush border of enterocytes and pancreatic alpha-amylase hydrolyze polysaccharides, oligosaccharides, and disaccharides into the constituent monosaccharides, namely, glucose, galactose and fructose. This is followed by absorption of monosaccharides.[8]
The α-(1→2) glycosidic bond of sucrose is hydrolyzed in a reaction catalyzed by sucrase-isomaltase (EC 3.2.1.48), an enzyme with two active sites, coded by a single gene.
One active site, the sucrase, hydrolyzes of the glycosidic bond of:
- sucrose, to yield glucose and fructose;
- some branched starches and short α-(1→4) linked glucose oligomers, with up to six glucose units, to yield glucose units;
- maltose, to yield two glucose units.
Note that this active site is responsible of about 80 percent of small bowel maltase activity.
The other active site, the isomaltase, catalyzes the release of straight chains from α-limit dextrins, glucose polymers with at least one α-(1→6) glycosidic bond, in an α-(1→6) glycosidase reaction.[3] Due to this activity, the enzyme is also called α-dextrinase.
Sucrase-isomaltase deficiency
Primary or congenital sucrase-isomaltase deficiency is a genetic disorder first reported in 1960.[14]
It is caused by more than twenty five mutations within the sucrase gene, and of which seven phenotypes are known. Either sucrase or isomaltase subunits can be affected.[3]
Since there are no membrane transporters for disaccharides, unabsorbed sucrose can cause osmotic diarrhea and, once it enters the colon, be partly metabolized by gut microbiota, which is part of the larger human microbiota. This leads to an excessive production of gas, such as methane, carbon dioxide and hydrogen, and short-chain fatty acids, mainly acetic acid, propionic acid, and butyric acid, and causes a great disconfort and osmotic-fermentative diarrhea.
The only treatment is to reduce or avoid dietary sucrose.[4]
Note: osmotic diarrhea is a consequence of the accumulation in the lumen of the distal portion of the small intestine and colon of non-absorbable and osmotically active solutes. For example, this occurs as a result of the deficiency of one or more disaccharidases of the brush border of enterocytes, which leads to disaccharide accumulation and to the consequent increase in osmotic pressure. In turn, this causes fluid to rush into the intestinal lumen leading to an excessive loss of electrolytes and fluids with the stool.
References
- ^ Albi T., Ruiz M.T., de los Reyes P., Valverde F., Romero J.M. Characterization of the sucrose phosphate phosphatase (SPP) isoforms from Arabidopsis thaliana and role of the S6PPc domain in dimerization. PLoS ONE 2016:11(11):e0166308. doi:10.1371/journal.pone.0166308
- ^ a b c Belitz H.-D., Grosch W., Schieberle P. Food Chemistry. 4th Edition. Springer, 2009
- ^ a b Cohen S.A. The clinical consequences of sucrase-isomaltase deficiency. Mol Cell Pediatr 2016;3(1):5. doi: 10.1186/s40348-015-0028-0
- ^ Grand R.J., Montgomery R.K., Chitkara D.K., Büller H.A. Carbohydrate and lactose malabsorption. Editor: Leonard R. Johnson. Encyclopedia of Gastroenterology. Elsevier, 2004, pag. 268-274. doi:10.1016/B0-12-386860-2/00103-9
- ^ a b c Heldt H-W. Plant biochemistry – 3th Edition. Elsevier Academic Press, 2005
- ^ Lunn J.E., Delorge I., Figueroa C.M., Van Dijck P., Stitt M. Trehalose metabolism in plants. Plant J 2014;79(4):544-67. doi:10.1111/tpj.12509
- ^ National Center for Biotechnology Information. PubChem Compound Summary for CID 5988, Sucrose. https://pubchem.ncbi.nlm.nih.gov/compound/Sucrose. Accessed Nov. 18, 2023.
- ^ a b Nelson D.L., M. M. Cox M.M. Lehninger. Principles of biochemistry. 6th Edition. W.H. Freeman and Company, 2012
- ^ Orna M.V., Eggleston, G. and Bopp, A.F. Chemistry’s role in food production and sustainability: past and present. Volume 1314 of ACS symposium series. American Chemical Society, 2019.
- ^ a b Ruan Y.L. Sucrose metabolism: gateway to diverse carbon use and sugar signaling. Annu Rev Plant Biol 2014;65:33-67. doi:10.1146/annurev-arplant-050213-040251
- ^ a b Stein O., Granot D. An overview of sucrose synthases in plants. Front Plant Sci 2019;10:95. doi:10.3389/fpls.2019.00095
- ^ a b United States Environmental Protection Agency (EPA). Sugar beet processing.
https://www.epa.gov/sites/default/files/2020-10/documents/b9s10-1b.pdf - ^ a b United States Environmental Protection Agency (EPA). Sugarcane processing. https://www3.epa.gov/ttnchie1/ap42/ch09/bgdocs/b9s10-1a.pdf
- ^ Weijers H.A., va de Kamer J.H., Mossel D.A., Dicke W.K. Diarrhoea caused by deficiency of sugar-splitting enzymes. Lancet. 1960;2(7145):296-7. doi:10.1016/s0140-6736(60)91381-7