Arachidonic acid or ARA or 20:4n-6 is a polyunsaturated fatty acid with a chain of 20 carbon atoms and 4 cis double bonds at positions 5, 8, 11 and 14. Since the first double bond, with respect to the methyl end, is at carbon 6, the molecule belongs to the group of omega-6 polyunsaturated fatty acids (PUFAs) or omega-6 fatty acids.
Like eicosapentaenoic acid or EPA or 20:5n-3, and docosahexaenoic acid or DHA or 22:6n-3, two omega-3 polyunsaturated fatty acids, arachidonic acid is a long-chain polyunsaturated fatty acid or LC-PUFA.
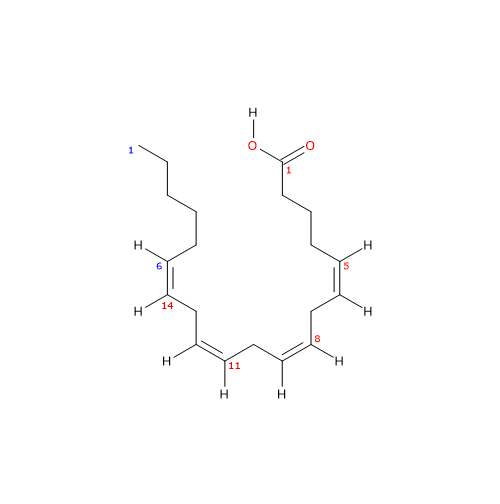
It is found mainly in cell membranes, esterified in phospholipids; hence, again like EPA and DHA, it is a structural components of cellular membranes. The highest content, expressed as a percentage of phospholipid fatty acids, is found in platelets.
Animals can synthesize arachidonic acid from linoleic acid or LA or 18:2n-6, the precursor to omega-6 polyunsaturated fatty acids, and, with alpha-linolenic acid or ALA or 18:3n-3, one of the two essential fatty acids for animals. In the absence of dietary linoleic acid, the other omega-6 polyunsaturated fatty acids, including ARA, become essential, too. For this reason, they are defined as conditionally essential fatty acids.
The elongases and desaturases that catalyze the conversion of linoleic acid to arachidonic acid and beyond, up to 22:5n6, are shared with the pathways leading to the synthesis of omega-3, omega-7 and omega-9 polyunsaturated fatty acids. Omega-3 polyunsaturated fatty acids seem to be the preferred substrates for desaturases, although the synthesis of omega-6 polyunsaturated fatty acids is prevalent due to the high dietary intake of linoleic acid.
Arachidonic acid is the precursor of many bioactive lipid mediators, molecules that appear to be responsible for many of the functional effects attributed to ARA. Examples are eicosanoids, namely, leukotrienes, prostaglandins, prostacyclins and thromboxanes, some with anti-inflammatory effects and others with pro-inflammatory effects.
The major dietary sources of arachidonic acid are eggs, meat and fish.
PROPERTIES, NAMES, AND IDENTIFIERS
Molecular weight: 304.467 g/mol
Molecular formula: C20H32O2
Melting point at -49.5 °C (-57.1 °F; 223.6 K)
Boiling point at 170 °C (338 °F; 443.1 K) at 0.15 mm Hg
IUPAC name:(5Z,8Z,11Z,14Z)-icosa-5,8,11,14-tetraenoic acid
CAS registry number: 506-32-1
PubChem: 444899
European Community (EC) Number: 208-033-4
Contents
- Synthesis
- Cellular distribution
- Arachidonic acid derivatives
- Arachidonic acid: harmful or helpful?
- Food sources
- References
Synthesis
Arachidonic acid can be synthesized from endogenous precursors, particularly linoleic acid or LA or 18:2n-6, the main PUFA in the Western diet.
Its synthesis, which occurs in the endoplasmic reticulum, involves five reactions, of which two desaturation steps and an elongation step.
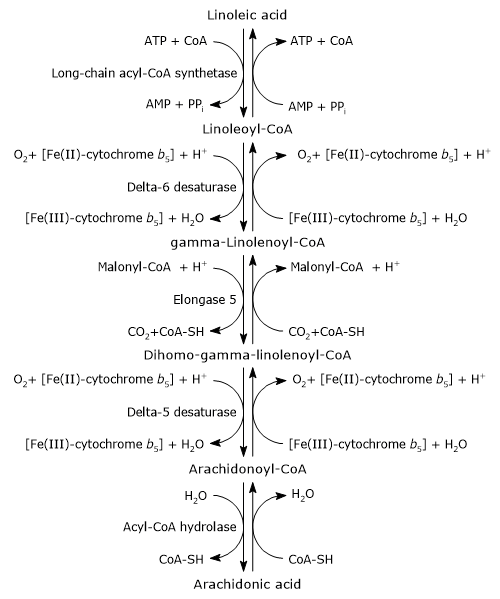
In the first step of the metabolic pathway, linoleic acid is activated by being joined to coenzyme A (CoA-SH). The reaction is catalyzed by a long-chain acyl-CoA synthetase (EC 6.2.1.3), at the expense of one molecule of ATP.
Linoleic acid + ATP + CoA ⇄ Linoleoyl-CoA + AMP + PPi
Linoleoyl-CoA is desaturated to gamma-Linolenoyl-CoA. The reaction is catalyzed by delta-6 desaturase (EC 1.14.19.3). The enzyme has a cytochrome b5 domain acting as the electron donor, and introduces a cis double bond at carbon 6, from the carboxyl end, of acyl-CoAs.
Linoleoyl-CoA + O2 + [Fe(II)-cytochrome b5] + H+ ⇄ gamma-Linolenoyl-CoA + [Fe(III)-cytochrome b5] + H2O
Delta-6 desaturase is the rate-limiting enzyme of the pathway and is affected by nutritional deficiencies as well as inflammatory processes.
Gamma-linolenoyl-CoA is elongated by two carbon atoms, namely an acetyl groups, to form dihomo-gamma-linolenoyl-CoA. The reaction is catalyzed by elongase 5 or elongation of very long chain fatty acids protein 5 (EC 2.3.1.199), and malonyl-CoA is the donor of the acetyl group.
Linolenoyl-CoA + Malonyl-CoA + H+ ⇄ Dihomo-gamma-linolenoyl-CoA + CO2 + CoA-SH
Dihomo-gamma-linolenoyl-CoA is desaturated to arachidonoyl-CoA. The reaction is catalyzed by delta-5 desaturase or acyl-CoA (8-3)-desaturase (EC 1.14.19.44) that, like delta-6 desaturase, contains a cytochrome b5 domain, and introduces a cis double bond at carbon 5 of acyl-CoAs containing a double bond at position 8 from the carboxyl end.
Dihomo-gamma-linolenoyl-CoA + O2 + [Fe(II)-cytochrome b5] + H+ ⇄ Arachidonoyl-CoA + [Fe(III)-cytochrome b5] + H2O
Delta-5 desaturase was suggested to be potentially rate-limiting during supplementation with gamma-linolenic acid, so much so that most of the dihomo-gamma-linolenic acid formed in the previous reaction is inserted at the sn-2 position of phospholipids, like arachidonic acid. Its activity is influenced by nutritional and environmental factors.
In the last step, the thioester bond of arachidonoyl-CoA is hydrolyzed with the release of arachidonic acid and coenzyme A. The reaction is catalyzed by an acyl-CoA hydrolase (EC 3.1.2.20).
Arachidonoyl-CoA + H2O ⇄ Arachidonic acid + CoA-SH
Based on animal studies, it has been assumed that in humans, increasing the intake of linoleic acid, the synthesis of ARA could increase. For this reason, it has been recommended to limit the intake of dietary LA to reduce tissue levels of ARA (see below).
However, it has been shown that there was no dose-response effect between the intake of dietary linoleic acid and ARA tissue levels in subjects consuming a Western-type diet. These data are also supported by the observation that, in adults, the rate of conversion of plasma/serum linoleic acid to ARA is very low, being between 0.3 percent and 0.6 percent. But why?
It seems that the limiting factor is not the saturation of tissue arachidonic acid content, but the reaction catalyzed by delta-6 desaturase, since, for example, the levels of ARA in blood phospholipids increase as a result of administration of gamma-linolenic acid and ARA itself.
Cellular distribution
Mammalian cells and tissues are rich in ARA, where it is found mainly in membrane phospholipids, mainly esterified in the sn-2 position. Arachidonic acid is arguably the most important among unsaturated fatty acids found in cell membranes.
In adult humans following a Western diet, its content varies in the different cell types. The highest values are found in platelets, where it constitutes about 25% of the phospholipid fatty acids. Lower values are found in mononuclear cells, liver, erythrocytes, skeletal muscle, neutrophils, and cardiac muscle, about 22, 20, 17, 17, 15 and 9 percent of the phospholipid fatty acids, respectively.
Arachidonic acid derivatives
The analysis of the metabolome of ARA highlights the presence of a constellation of metabolites, such as PUFAs with higher degree of unsaturation and longer carbon chain, as well as many bioactive lipids, some with pro-inflammatory activities, and others with anti-inflammatory activities or able to promote the resolution of inflammatory injuries.
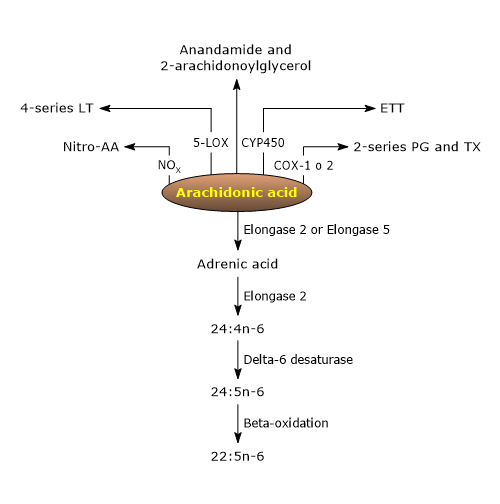
Among these bioactive lipid mediators there are ligands for endocannabinoid receptors, such as anandamide and 2-arachidonoylglycerol, nitrated arachidonic acid, which is an inflammatory and vascular signaling molecule, but especially eicosanoids.
Eicosanoids
Eicosanoids, mediators with autocrine and paracrine activity, are a group of oxygenated 20 carbon fatty acids. Their main precursor is arachidonic acid; others are dihomo-gamma-linolenic acid, EPA and DHA.
Their biosynthesis, like that of the other bioactive lipid compounds seen previously, is preceded by the release of ARA from membrane phospholipids in a reaction catalyzed by phospholipase A2 (EC 3.1.1.4). A second, minor, pathway for the release of ARA is the hydrolysis of diacylglycerol or DAG, in a reaction catalyzed by DAG lipase (EC 3.1.1.-).
The pathways leading to the biosynthesis of eicosanoids from arachidonic acid are known as “arachidonate cascade”, and three major pathways may be identified, which are named from the enzyme that catalyzes the first step.
- The cyclooxygenase pathway, in which the first reaction is catalyzed by prostaglandin-endoperoxide synthase or PTGS or cyclooxygenase (EC 1.14.99.1).
There are two cyclooxygenase isoenzymes, referred to as 1, and 2, or COX-1 and COX-2, and also known as PTGS1 and PTGS2, and prostaglandin G/H synthase 1 and 2.
COX-1 is the constitutive enzyme and is present in most cells, except red blood cells.
COX-2 is the inducible form. It is constitutively expressed in uninflamed tissues and organs, such as heart, kidney, the vasculature, gastric epithelium and brain, but may be induced by inflammatory stimuli in epithelial cells, white blood cells, and smooth muscle cells.
This pathway leads to the synthesis of 2-series prostaglandins (PT), and their derivatives 2-series thromboxanes (TX), so called because they have two double carbon-carbon bonds in their side chains.
Some of these molecules have pro-inflammatory activity, such as prostaglandin E2 or PGE2, others anti-inflammatory activity, such as thromboxane A2 or TXA2. - The lipoxygenase pathway, in which the first reaction is catalyzed by the arachidonate 5-lipoxygenase or 5-lipoxygenase o 5-LOX (EC 1.13.11.34). This reaction leads to the formation of 5(S)-hydroperoxy eicosatetraenoic acid or 5S-HPETE.
This pathway leads to the synthesis of 4-series leukotrienes (LT), so called because they have four double carbon-carbon bonds in their side chains.
LT are pro-inflammatory signaling molecules, such as leukotriene B4 or LTB4, and are the precursors of the lipoxins (LX), eicosanoids with anti-inflammatory properties, such as lipoxin A4 or LXA4 and lipoxin B4 or LXB4. - The epoxygenase pathway, in which the first reaction is catalyzed by a cytochrome P450 epoxygenase.
This pathway leads to the synthesis of epoxyeicosatrienoic acids or EETs, molecules with vasorelaxant activity, profibrinolytic as well as anti-inflammatory effects. In vivo, EETs are rapidly metabolized to dihydroxyeicosatrienoic acids or DHETs, in reactions catalyzed by soluble epoxy hydrolase. DHETs are less active than EETs.
Two other series of reactions are grouped into the “epoxygenase pathway”. They are initiated by other cytochrome P450 mixed-function oxidases. These enzymes lead to the synthesis of hydroperoxyeicosatetraenoic acids or HPETEs, some of which are epimers of lipoxygenase catalyzed products, and omega-oxidized monohydroxyeicosatetraenoic acids.
Omega-6 PUFA derivatives
Arachidonic acid can be converted into 22:5n-6, through four reactions, of which the first three occur in the endoplasmic reticulum, whereas the last one in peroxisomes.
- In the first step, ARA is elongated to form adrenic acid or 22:4n-6, in the reaction catalyzed by elongase 2 or Elovl2 (EC 2.3.1.199) or elongase 5 or Elovl5.
- Adrenic acid is elongated to 24:4n-6, in the reaction catalyzed by elongase 2.
- 24:4n-6 is desaturated to 24:5n-6, in the reaction catalyzed by delta-6 desaturase.
- In the last step, 24:5n-6 undergoes peroxisomal beta-oxidation, to form 22:5n-6.
Arachidonic acid: harmful or helpful?
The idea that arachidonic acid may be harmful is derived from the following observations.
- Many ARA-derived mediators are involved in many pathologies, having a pro-inflammatory action.
- Free arachidonic acid itself is an immunosuppressant, induces inflammatory responses, and is a potent platelet aggregator.
- Many health benefits of omega-3 polyunsaturated fatty acids involve an “antagonism” of ARA: often, in fact, they partly replace it in membrane phospholipids and inhibit its metabolism to eicosanoids.
- A study published in 1975, in which 6 g/day of ARA were administered to healthy volunteers, was stopped because of a marked increase in ex vivo platelet aggregation, a pro-thrombotic event.
However, it is important to keep in mind that the aforementioned effects were only observed in certain tissues or cells, and often under non-physiological conditions, such as the study conducted in 1975. Therefore these effects, as their effectiveness under physiological conditions, remain to be defined.
Conversely, in subjects receiving 1.5 g/day of arachidonic acid, no effects have been observed on inflammatory markers, a range of immune functions, platelet reactivity, and bleeding time. All this indicates that ARA intake up to 1.5 g/day by healthy adults does not appear to have any adverse effects.
Finally, it should be emphasized that infant formulas containing ARA in combination with DHA are associated with improved growth and development, and in premature infants, with a development of the immune system similar to that of breastfed infants, reducing also the risk of necrotizing enterocolitis. Therefore, ARA seems to have an important role for the proper growth and development of infants.
Food sources
Important dietary sources of arachidonic acid are listed below.
- Meat.
It is found both in lean cuts, where it is concentrated in phospholipids, and in visible fat.
The highest concentration is found in pork fat, for example about 11 mg/g in lard, whereas among lean cuts the richest source is duck.
Lamb and beef meat, both lean cuts and visible fat, are those that contain the lowest levels. - Eggs.
- Fish.
For example about 3 mg/g in tuna and salmon, and 0.4 mg/g in herring.
The average daily intake for an adult following a Western diet is estimated to be between 50 and 300 mg.
References
- Akoh C.C. and Min D.B. Food lipids: chemistry, nutrition, and biotechnology. 3th Edition. CRC Press, Taylor & Francis Group, 2008
- Calder P. C. Dietary arachidonic acid: harmful, harmless or helpful? Br J Nutr 2007; 98:451-453. doi:10.1017/S0007114507761779
- Chow Ching K. Fatty acids in foods and their health implication. 3th Edition. CRC Press, Taylor & Francis Group, 2008
- Farvid M.S., Ding M., Pan A., Sun Q., Chiuve S.E., Steffen L.N., Willett W.C., Hu F.B. Dietary linoleic acid and risk of coronary heart disease: a systematic review and meta-analysis of prospective cohort studies. Circulation 2014;130:1568-1578. doi:10.1161/CIRCULATIONAHA.114.010236
- Gregory M.K., Gibson R.A., Cook-Johnson R.J., Cleland L.G., James M.J. Elongase reactions as control points in long-chain polyunsaturated fatty acid synthesis. PLoS One 2011;6(12):e29662. doi:10.1371/journal.pone.0029662
- Harris W.S., Shearer G.C. Omega-6 fatty acids and cardiovascular disease. Friend, not foe? Circulation 2014;130:1562-1564. doi:10.1161/CIRCULATIONAHA.114.012534
- Lee J.M., Lee H., Kang S. 3 and Park W.J. Fatty acid desaturases, polyunsaturated fatty acid regulation, and biotechnological advances. Nutrients 2016;8(1):23. doi:10.3390/nu8010023
- Li D., Ng A, Mann N.J., Sinclair A.J. Contribution of meat fat to dietary arachidonic acid. Lipids 1998;33(4):437-440. doi:10.1007/s11745-998-0225-7
- Rett B.S. and Whelan J. Increasing dietary linoleic acid does not increase tissue arachidonic acid content in adults consuming Western-type diets: a systematic review. Nutr Metab (Lond) 2011;8:36. doi:10.1186/1743-7075-8-36
- Seyberth H.W., Oelz O., Kennedy T., Sweetman B.J., Danon A., Frolich J.C., Heimberg M. and Oates J.A. Increased arachidonate in lipids after administration to man: effects on prostaglandin biosynthesis. Clin Pharmacol Ther 1975;18:521-529. doi:10.1002/cpt1975185part1521