Lipid absorption in the small intestine involves the digestion products of triglycerides, phospholipids, cholesterol esters, and fat-soluble vitamin esters, that is, free fatty acids, small amounts of 2-monoglycerides, lysophospholipids, mainly lysophosphatidylcholine, cholesterol, fat-soluble vitamins and glycerol. These molecules, with the exception of short-chain fatty acids, such as acetic acid, butyric acid, and propionic acid, and medium-chain fatty acids, namely, caproic acid, caprylic acid, capric acid, and lauric acid, and glycerol, have a poor solubility in aqueous medium.
Contents
- Mixed micelles and lipid absorption
- Absorption of long chain fatty acids
- Absorption of 2-monoglycerides
- Absorption of cholesterol
- References
Mixed micelles and lipid absorption
The luminal surface of enterocytes is covered by a layer of water called “unstirred water layer”, whose thickness depends inversely on how vigorously the luminal content has been mixed, and which represents the main barrier that the less soluble lipids must cross to be absorbed.
The water barrier is overcome by the formation of mixed micelles that are made up of bile salts (pure micelles consist of only bile salts), and products of lipid digestion. Their formation begins as the pH of luminal content rises, and the concentration of bile salts reaches or exceeds the “critical micellar concentration”, a value that in the intestinal lumen is almost always exceeded.
These micelles have a structure similar to a membrane bilayer discs cut out from a biological membrane, with size ranging from 40 to 600 Å, therefore, much smaller than emulsified lipid droplets. Bile salts stabilize the cut edges, that is, their hydrophobic face is oriented towards the centre of disk, and the hydrophilic portion faces the external environment.
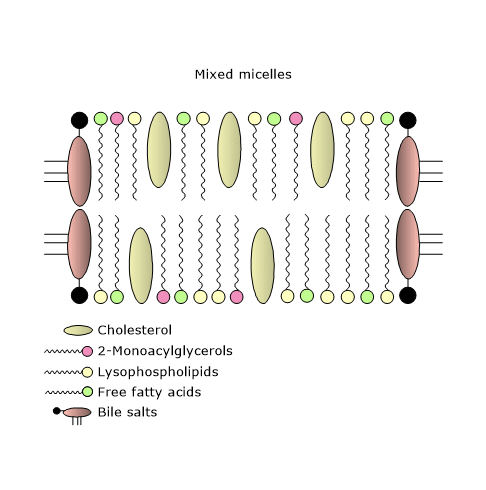
Therefore, bile salts allow the formation of water-soluble micelles, which transport the less soluble lipids to the apical surface of the enterocytes. And thanks to the mixed micelles, the concentration of the fatty acids, 2-monoacylglycerols, lysophospholipids, free cholesterol, the only form of cholesterol that can be incorporated into these micelles and then absorbed from the small intestine, and fat-soluble vitamins increases close to the brush border membrane by a factor of 100 to 1000.
The concentration gradient of the products of lipid digestion between the outside and the inside of the cell, along which these molecules flow, is maintained thanks to their rapid re-esterification to triglycerides, cholesterol esters and phospholipids inside the cell.
Calcium ion, present in relatively high concentrations in both pancreatic juice and bile, aids the absorption of lipid digestion products at the water-lipid interface.
Finally, it is interesting to note that unlike carbohydrates, which are absorbed after hydrolysis to the monosaccharides glucose, fructose and galactose, lipid absorption involves both free fatty acids and non-esterified cholesterol, and partially hydrolysed lipids, such as 2-monoacylglycerols and lysophospholipids.
Note: the proper absorption of fat-soluble vitamins depends on an adequate intake of lipids.
Absorption of long chain fatty acids
The absorption of long chain fatty acids occurs both by passive diffusion and by facilitated transport, also known as facilitated diffusion, by specific protein carriers.
- Passive diffusion occurs via a flip-flop mechanism, and affects protonated, and thus uncharged, fatty acids.
- Protein mediated transfer of long chain fatty acids may not be quantitatively important, but it seems to play a regulatory role on the subsequent metabolic fate of absorbed fatty acids.
Once inside the enterocyte, fatty acids are trapped by binding to fatty acid binding proteins and/or by their conversion to the corresponding acyl-CoA.
Two proteins involved in their absorption are CD36 and FATP4.
Note: short-chain and medium chain fatty acids are more soluble than long chain fatty acids. They are absorbed and enter directly the portal system in which they are carried by albumin.
Cd36
CD36 is a highly glycosylated integral membrane protein with a broad ligand specificity, being able to bind native lipoproteins and modified lipoproteins such as oxidized LDL, long-chain fatty acids, glycosylated proteins, malaria-infected erythrocytes, amyloid B, collagen, and thrombospondin-1.
It is expressed ubiquitously, for example in the adipose tissue, heart, skeletal muscle, capillary endothelium, and small intestine in which it is abundantly expressed on the brush border membrane of the enterocytes of duodenum and jejunum. It seems to be absent in the ileum and colon, whereas it is present in blood vessels throughout the intestine.
The contribution of CD36 to fatty acid absorption seems modest; in fact, after excessive lipid ingestion a shift of their absorption to more distal parts of the intestine occurs. Its small contribution is due at least to two reasons.
- The Km for fatty acid absorption is in the nanomolar range. Therefore, it may have a role in the very early stages of digestion process, and then be rapidly saturated.
- It seems that fatty acids down-regulate protein levels by promoting its ubiquitination, which occurs in muscle and liver as well.
Instead, many studies suggest that its main role may be to optimize, in the very early stages of lipid absorption, the events that facilitate the assembly of fatty acids and cholesterol, absorbed in the proximal small intestine, into chylomicrons.
The protein also appears to play a role at other steps of lipid digestion and lipid absorption. At oral level, it mediates fat taste perception in taste buds of the tongue, in the circumvallate papillae, and the start of the cephalic phase of digestion. In the small intestine, it provides oleic acid molecules required for the synthesis of oleoylethanolamide or OEA, which is involved in satiety regulation.
FATP4
It is a member of very long chain acyl-CoA synthetase family (EC 6.2.1.3; there are 13 long-chain acyl-CoA synthetase isoforms), also called fatty acid transport proteins or FATPs.
FATP4, highly expressed in mammalian enterocytes, is not a brush border membrane protein, but is localized in the subapical membranes and endoplasmic reticulum. In these subcellular locations, it indirectly participates in fatty acid uptake through their esterification with CoA to form acyl-CoA. The newly synthesized acyl-CoAs are rapidly converted to di- and triglycerides and phospholipids. However, experiments with genetically modified mice have shown that the role of FATP4 in lipid absorption seems dispensable.
Absorption of 2-monoglycerides
Studies in cell lines suggest that a membrane protein is involved in their uptake, since it is a saturable function of their concentration and exhibits sensitivity to trypsin digestion.
It has been observed that 2-monoglycerides inhibit fatty acid uptake, which suggests that their transport and that of fatty acids may be coordinated to optimize triacylglycerol resynthesis inside the enterocyte. The observation that their metabolic fate, like that of fatty acids, depends upon the site of entry into the enterocyte further support this hypothesis. In fact, experiments in rats and mice showed that 2-monoglycerides absorbed across the apical or the basolateral surface have a different metabolic fate.
- The ratio of triglyceride to phospholipid formed from 2-monoglycerides is about 10-fold greater for apical than basolateral delivery, and similar observations were made with fatty acids.
- It was observed a fatty acid incorporation 2-fold higher in phosphatidylethanolamine, and a 3-fold reduction in the ratio of phosphatidylcholine to phosphatidylethanolamine for the apical compared with basolateral uptake. And this, as mentioned above, suggests that the proteins of the apical membrane facilitate fatty acid transfer, and direct these molecules to specific metabolic sites.
- Further, the level of fatty acid oxidation is 3-fold greater for basolateral delivery.
Absorption of cholesterol
Intestinal cholesterol homeostasis is the result of a complex balance between its absorption, de novo synthesis, daily losses due to the sloughed enterocytes, and transport by lipoproteins, mainly chylomicrons.
Below, you will examine a protein involved in the interaction between cholesterol and brush border membrane of the enterocyte: NPC1L1.
NPC1L1
It is a member of the Niemann-Pick C1 (NPC1) family of lipid transport facilitators. NPC1 is involved in the egress of cholesterol from the lysosomes to the plasma membrane and the endoplasmic reticulum.
They are membrane proteins containing 13 transmembrane domains; five of these, from 3 to 7, aggregate to form a sterol-sensing domain or SSD, which is also found in other proteins involved in cholesterol homeostasis, such as HMG-CoA reductase (EC 1.1.1.34), the key enzyme in the de novo synthesis of the steroid, or 7-dehydrocholesterol reductase (EC 1.3.1.21).
The protein, highly expressed in the small intestine, where it is localized on the brush border membrane of the enterocyte (and in the liver, in the canalicular membrane of the hepatocytes) mediates the absorption of dietary cholesterol and the reabsorption of biliary cholesterol through vesicular endocytosis. Finally, its activity seems also to be influenced by glycosylation.
Its cellular localization is regulated by cholesterol; in fact, it moves from the endocytic recycling compartment, which is part of an endosomal system involved in the recycling of many transmembrane proteins, to the plasma membrane in response to changes in cholesterol levels.
When cholesterol level is low, the transport of the protein to the plasma membrane is activated.
But when cholesterol binds to the SSD domain of the protein, a conformational change may occur, which in turn triggers its vesicular internalization, mediated by clathrin-AP2 complex, together with cholesterol itself and flottilin-1 and flottilin-2, proteins of lipid rafts that form a complex with NPC1L1.
NPC1L1 might therefore provide another point for the regulation of cholesterol homeostasis, in addition to the feedback inhibition of HMG-CoA reductase and LDL receptor.
Once NCP1L1 and bound sterols, that is, cholesterol, sterols of plant origin such as sitosterol, campesterol and sitostanol, and brassicasterol, the main sterol of shellfish, are transported to the endoplasmic reticulum, they esterified by acyl-CoA:cholesterol acyltransferase 2 or ACAT2 (EC 2.3.1.26).
MyoVb•Rab11a•Rab11-FIP2 triple complex
The transport of NPC1L1 to the plasma membrane depends on actin microfilaments and MyoVb•Rab11a•Rab11-FIP2 triple complex, the latter essential for the endocytic vesicular transport and the recycling of many other proteins, such as GLUT4 , CFTR, beta2 adrenergic receptors, and transferrin receptor.
Which are the components of the triple complex?
- MyoVb belongs to the family of Myosins, motor proteins responsible for actin based mobility. Myosins Va, Vb, and Vc are involved in a wide range of events of vesicular transport in different mammalian tissues.
- Rab11a belongs to the family of small GTPases (EC 3.6.5.2), and recruits MyoVb to their target vesicles.
- Rab11 family-interacting protein 2 (class I) or Rab11-FIP2 is an adapter protein that bridges the interactions between MyoVb and the proteins of endocytic vesicle recycling.
How does the triple-complex work?
At steady-state, Rab11a and Rab11-FIP2 bind to NPC1L1, all located on the membranes of the endocytic recycling system, and mediate the interaction of NCP1L1 with MyoVb.
When cellular cholesterol levels fall, Rab11a•Rab11-FIP2 dissociates from NPC1L1-MyoVb complex, which allows the export of the complex to the plasma membrane in a microfilament-dependent manner. At the same time, a protein called Cdc42 is activated and binds to NPC1L1-MyoVb complex. Then, the budding of the vesicles is initiated, and this allows the export of the complex out of the endocytic recycling system and its migration to the plasma membrane.
Once in the plasma membrane, MyoVb and actin dissociate from NPC1L1.
Cdc42
It is a member of Rho family of small GTPases, and appears to be essential in the regulation of intracellular trafficking of NPC1L1 as a result of changes in intracellular cholesterol levels. Moreover, many functions have been ascribed to it, both in cell polarity and membrane trafficking.
The protein switches between a GTP-bound active state to a GDP-bound inactive state as a result of cholesterol depletion. The switch between the two states is regulated by several factors, such as:
- guanine nucleotide exchange factors or GEFs;
- guanine nucleotide dissociation inhibitors or GDIs;
- GTPase-activating proteins or GAPs;
Because specific GEFs generally mediate the activation of Cdc42, cholesterol depletion may cause change in membrane architecture, with spatial rearrangement of the proteins which may lead to the contact of Cdc42 and GEFs, and the following activation of the small GTPase.
Once bound to GTP, the protein interacts with N-WASP, activating it; N-WASP in turn binds and activates Arp2/3 complex which initiates the branched polymerization of actin microfilaments, essential for the formation and transport of vesicles.
How does Cdc42 play an important role in lipid absorption?
Cholesterol depletion activates Cdc42, and the binding of Cdc42-GTP to NPC1L1 promotes the dissociation of Rab11a and the association of MyoVb and actin.
Cdc42-GTP complex can also activate N-WASP-Arp2/3 complex, that regulates actin polymerization close to NPC1L1 containing vesicles. Actin microfilaments may in turn drive vesicle budding and scission, and finally their movement.
Therefore, the events related to the vesicular trafficking are dependent on motor proteins and the cytoskeleton.
Influences on the absorption of dietary cholesterol
As previously seen, mixed micelles are essential for the intestinal absorption of cholesterol. However, about 50 percent of the dietary cholesterol is lost in the stools. Different factors affect these percentages, such as the fibers, the intestinal transit time, the total amount of cholesterol present in small intestinal lumen, as well as the more polar lipids.
- A high fiber diet reduces the absorption of cholesterol by at least two factors.
Fibers such as lignin and cellulose reduce its availability, both acting as adsorbents and reducing the residence time of food in the small intestine, and therefore the time available for absorption.
Gums and pectins work slightly differently, acting not as adsorbents, but conferring luminal content a gel-like consistency that makes cholesterol less available for absorption. These fibers reduce the intestinal transit time as well. - The absorption of the more polar lipids, which together with bile salts are needed to form mixed micelles, can cause precipitation of cholesterol, thus rendering the molecule unavailable for absorption.
- Finally, the greater the amount of cholesterol present and the smaller will be the amount absorbed, and vice versa.
It should be noted that the rate of cholesterol absorption in the small intestine is very low compared to that of acylglycerols and fatty acids.
References
- Abumrad N.A. and Davidson N.O. Role of the gut in lipid homeostasis. Physiol Rev 2012:92(3);1061-1085. doi:10.1152/physrev.00019.2011
- Berdanier C.D., Dwyer J., Feldman E.B. Handbook of nutrition and food. 2th Edition. CRC Press. Taylor & Francis Group, 2007
- Chu B-B., Ge L., Xie C., Zhao Y., Miao H-H., Wang CJ., Li B-L., and Song B-L. Requirement of Myosin Vb·Rab11a·Rab11-FIP2 complex in cholesterol-regulated translocation of NPC1L1 to the cell surface. J Biol Chem 2009:284(33);22481-22490. doi:10.1074/jbc.M109.034355
- Rosenthal M.D., Glew R.H. Medical biochemistry – Human metabolism in health and disease. John Wiley J. & Sons, Inc., Publication, 2009
- Skov M., Tonnesen C.K., Hansen G.H., Danielsen E.M. Dietary cholesterol induces trafficking of intestinal Niemann-Pick Type C1 Like 1 from the brush border to endosomes. Am J Physiol Gastrointest Liver Physiol 2011:300(1);G33–G40. doi:10.1152/ajpgi.00344.2010
- Stipanuk M.H., Caudill M.A. Biochemical, physiological, and molecular aspects of human nutrition. 3rd Edition. Elsevier health sciences, 2012
- Storch J., Zhou Y.X., Lagakos W.S. Metabolism of apical versus basolateral sn-2-monoacylglycerol and fatty acids in rodent small intestine. J Lipid Res 2008;49(8):1762-1769. doi:10.1194/jlr.M800116-JLR200
- Xie C., Li N., Chen Z.J., Li B.L., Song B.L. The small GTPase Cdc42 interacts with Niemann-Pick C1-like 1 (NPC1L1) and controls its movement from endocytic recycling compartment to plasma membrane in a cholesterol-dependent manner. J Biol Chem 2011;286:35933-35942. doi:10.1074/jbc.M111.270199