The body requires proteins, and particularly the essential amino acids contained in them. The recommended daily intake for a healthy adult subject is 0.85 g/kg body weight, so for a 70 kg person is about 60 g, but often in the typical Western diet the intake is greater than 100 g/day. In addition to food proteins, protein digestion also involves 50-100 g of endogenous proteins secreted or lost into the lumen of the gastrointestinal tract, and which result from:
- saliva;
- gastric juice;
- pancreatic enzymes and other secretions;
- intestinal cells that flake off;
- proteins that pass into the intestinal lumen from the bloodstream.
This mixture is efficiently digested and absorbed in the duodenum, the first and the shortest part of the small intestine, with a daily loss through faeces equal to about 1.6 g of nitrogen, equivalent to 10 g of protein. Most of the lost nitrogen is used by the gut microbiota in the colon for its growth, and thus it is found in the faeces as part of the bacterial mass.
Contents
Digestive enzymes
Protein digestion occurs as a result of the hydrolysis of the peptide bonds that bind the individual amino acids in the polypeptide chain. These reactions are catalyzed by enzymes called proteases.
The intestinal proteases, specific for the amino acid side-chain, are hydrolases that can be divided into two classes:
- endopeptidases, which hydrolyze peptide bonds within the polypeptide chain, and are produced by the stomach and the exocrine pancreas;
- exopeptidases, that can be divided into two groups:
the carboxypeptidase, which remove amino acids from C-terminal end, and are produced by the exocrine pancreas;
the aminopeptidase, which act on the N-terminal end, and are produced by the enterocytes.
Site of synthesis | Zymogen | Active enzyme |
Stomach | Pepsinogen | Pepsin |
Pancreas | Chymotrypsinogen | Alpha-Chymotrypsin |
Pancreas | Trypsinogen | Trypsin |
Pancreas | Procarboxypeptidase A and B | Carboxypeptidase A and B |
Pancreas | Proelastase | Elastase |
Pancreas | Prolipase | Lipase |
These enzymes are synthesized and secreted in an inactive form, called zymogens or proenzymes.
Within the cell, zymogens are stored inside membrane-bounded granules called zymogen granules. When the cell is stimulated by the specific signal, the granule membrane fuses with the plasma membrane and zymogens are released by exocytosis.
These proteases are synthesized in an inactive form to avoid that, before being secreted, they digest themselves and/or tissue proteins. What happens is that the active site of the enzyme is “masked” and only after activation the protein can act on the substrate. The activation is the result of the cleavage, catalyzed by a specific enzyme, of one or more specific peptide bonds, with release of one or more segments of the polypeptide chain. This allows the molecule to assume a three-dimensional conformation in which the active site is free and properly configured.
Even their accumulation within the granules is a protection system: it isolates them from the other molecules present in the cell.
Most proteins in their native conformation are resistant to the action of proteases. This is a consequence of their secondary and tertiary or native structure that mask many bonds to the action of the enzymes. These structures are stabilized by covalent bonds, such as disulfide bridges between cysteine residues, and non-covalent forces, such as ionic interactions, hydrogen bonds and van der Waals forces.
For an adequate protein digestion it is therefore essential that peptide bonds are as accessible as possible to the action of the intestinal proteases. This is achieved, outside the body through the cooking of food, and within the body by the acid environment in the stomach.
Role of the cooking and chewing of food
The cooking of food, when it is not excessive, facilitates protein digestion.
In what way?
Like all molecules, also proteins are not motionless but vibrate. As the temperature increases the proteins vibrate at a greater amplitude, up to destroy the noncovalent bonds that contribute to the maintenance of the native structure. Therefore, a conformational change of the protein occurs, that is, the molecule is denatured. This can make internal peptide bonds more accessible to the action of digestive enzymes.
Even the chewing and insalivation of food homogenize and humidify the solid components of the food itself, facilitating the gastric and small intestinal digestion.
Phases
Two phases are involved in protein digestion: the first occurs in the stomach and the other in the in the duodenum, the first part of the small intestine.
Gastric phase
Protein digestion begins in the stomach, and this is a “preparation stage” compared to the events that occur in the duodenum.
The presence of food in the stomach stimulates G cells of the mucosa of the gastric antrum and proximal duodenum to produce and release the hormone gastrin into the bloodstream. The hormone stimulates the parietal cells of the proper gastric glands, localized mostly at the bottom of the organ, to produce and secrete hydrochloric acid into the stomach. Parietal cells also produce the intrinsic factor, a protein that binds vitamin B12, preventing its destruction and allowing it to be absorbed.
In the proper gastric glands you also found:
- mucous neck cells, that produce mucus;
- chief cells, that release pepsinogen.
All of these substances, together with others such as potassium ions and the gastric lipase, are present in the gastric juice, which has a pH that ranges between 1 and 2.5.
Due to its low pH, the gastric juice has an antiseptic action, killing most bacteria and other foreign cells, and a denaturing effect, as it breaks the noncovalent bonds that maintain the native structure of proteins. This denaturing effect facilitates the access of intestinal protease to peptide bonds, like the heating during cooking. Some proteins rich in disulfide bonds, such as keratins, are resistant to denaturation by low pH, and hence difficult to digest. On the contrary, most of the globular proteins are almost completely hydrolyzed into constituent amino acids.
Finally, the low pH of the gastric juice activates pepsinogen, a zymogen, to pepsin, the first enzyme involved in protein digestion.
Pepsin
There are different isoenzymes of pepsinogen, such as type I, synthesized by the cells of the body and fundus of the stomach, and type II that is produced in all the regions of the organ. All the isoenzymes are converted to the active enzyme. The activation occurs via autocatalysis, at pH values below 5, by an intramolecular process consisting in the hydrolysis of a specific peptide bond and release of a small peptide from the N-terminal end of the proenzyme. This peptide remains bound to the enzyme and continues to act as an inhibitor until the pH drops below 2, or until it is further degraded by pepsin itself. So, once some pepsin is formed, this quickly activates other pepsinogen molecules.
Pepsin, an endopeptidase with an optimum pH of activity at 1.6, hydrolyses 10-20% of the proteins in the meal. Many digestive enzymes are able to act on a wide range of substrates, and pepsin is no exception, catalyzing the cleavage of peptide bonds adjacent to amino acid residues such as leucine and phenylalanine, tyrosine and tryptophan, aromatic amino acids. A mixture of peptides of large size and a few free amino acid are produced.
Pepsin action is important not so much for its direct contribution to protein digestion, that is modest, but for the release of peptides and amino acids which, at the duodenal level, stimulate the secretion of cholecystokinin, and therefore the duodenal/pancreatic phase of protein digestion.
It should be noted that the action of pepsin on collagen, a family of proteins that wrap around and hold together the muscle cells, facilitates the access of the pancreatic protease to meal proteins.
Small intestinal phase
When the gastric content passes into the duodenum, its acidity stimulates S cells, localized in the duodenal mucosa and in the proximal part of the jejunum, the next part of the small intestine, to produce and release the hormone secretin into the bloodstream. The hormone causes the secretion of an alkaline pancreatic juice, rich in bicarbonate ions but poor in enzymes, which passes into the duodenum through the pancreatic duct. In the duodenum, it neutralizes the hydrochloric acid produced by the stomach, raising pH to around 7. Secretin also stimulates bile secretion and reduces gastrin release.
The presence of amino acids in the duodenum stimulates, as mentioned above, endocrine cells in the duodenum and jejunum to produce and release cholecystokinin (CKK) into the bloodstream. The hormone, among other functions, stimulates exocrine pancreas to secrete a juice rich in enzymes, present as zymogens, that is:
- trypsinogen, chymotrypsinogen and proelastase, endopeptidases with a different substrate specificity compared to pepsin but also among them;
- procarboxypeptidase A and B, exopeptidases which remove amino acids from the C-terminal end of the peptides.
Therefore, in the duodenum there is a neutral environment rich in enzymes able to continue, once activated, protein digestion. Moreover, as the proteases have different substrate specificity, each peptide produced by an enzyme can be substrate of another enzyme.
In pancreatic juice, alpha-amylase, lipase and nuclease are also present.
Activation of pancreatic zymogens
The first and master step in their activation is the conversion of trypsinogen to trypsin by enteropeptidase, also called enterokinase, an endopeptidase produced by cells of the duodenum after cholecystokinin stimulation. Enteropeptidase catalyses the cleavage of a specific peptide bond between a lysine residue and an isoleucine residue of the trypsinogen, with release of a hexapeptide. This causes a conformational rearrangement of the protein that activates it, that is, trypsin is formed.
The enzyme cleaves peptide bonds adjacent to lysine and arginine residues of protein to digest; moreover, it can activate chymotrypsinogen, proelastase and procarboxypeptidase A and B, but also other molecules of trypsinogen, like pepsin, via autocatalysis.
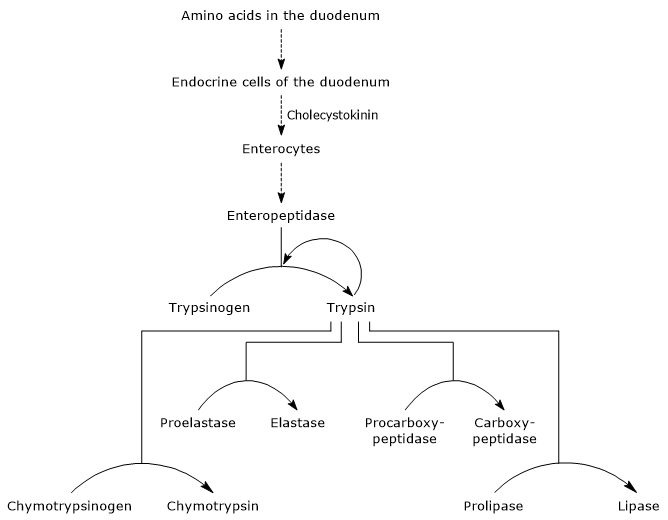
Therefore, the ability of the duodenum to digest proteins increases as the pancreatic zymogens are activated, all triggered by a small amount of enteropeptidase.
- The activation of chymotrypsinogen to chymotrypsin occurs through different steps to which trypsin and the activated chymotrypsin itself take part.
In the first step, trypsin catalyzes the cleavage of a specific peptide bond, and this causes the activation of chymotrypsinogen to pi-chymotrypsin, which is fully active. Then, pi-chymotrypsin itself catalyzes the release of two dipeptides with formation of the delta-chymotrypsin, a more stable form of the enzyme. delta-Chymotrypsin undergoes two conformational changes, the first of which leads to the formation of the kappa-chymotrypsin, and the second of alpha-chymotrypsin, the final active form of the enzyme.Activation of Chymotrypsinogen Chymotrypsin acts on peptide bonds adjacent to phenylalanine, tryptophan, methionine, tyrosine and leucine residues.
- Proelastase is activated to elastase by the removal of a small peptide from the N-terminal end.
Elastase, which is less specific than the other digestive hydrolases, catalyzes the cleavage of peptide bonds adjacent to amino acids such as glycine, alanine and serine. - Procarboxypeptidase
Procarboxypeptidase A is activated to carboxypeptidase A; the protease cleaves peptide bonds adjacent to amino acids with branched or aromatic side chains, such as phenylalanine and valine.
Procarboxypeptidase B is activated to carboxypeptidase B, specific for amino acids with basic side chains, such as lysine and arginine.
The above-mentioned proteases, unlike pepsin, have an optimum pH of action ranging from7 to 8, that is, neutral or weakly alkaline.
Pancreatic trypsin inhibitor
There is an “additional” protection system against intrapancreatic trypsin activity: the synthesis of an inhibitor called “pancreatic trypsin inhibitor”. The molecule, present in the pancreatic zymogen granules, is capable of binding very tightly the active site of the enzyme inactivating it. In this way, the activity of any trypsin resulting from a premature activation of trypsinogen is blocked, preventing a situation in which a few activated molecules activate all the pancreatic zymogens.
In plants, there are many molecules with similar activity. An example is the Kunitz trypsin inhibitor, a protein mainly found in soybeans, that forms a very stable complex with the active site of trypsin.
Since the above-mentioned proteases have a distinct substrate specificity, acting on peptide bonds adjacent to different amino acids, each peptide generated by a protease can be substrate of another one. In this way the partially digested proteins that enter the duodenum are efficiently hydrolyzed into free amino acids and peptides of 2-8 residues. These peptides are substrates of aminopeptidases secreted from enterocytes and associated with their microvilli.
Dipeptidase are also present.
It should be noted that the same proteases are finally digested, ending the process.
The importance and effectiveness of proteolytic enzymes in the intestinal protein digestion can be understood from this example. If in vitro you want to hydrolyze a protein into its constituent amino acids, it is necessary to use a strong and concentrated acid as a catalyst and to heat the sample at 105 °C overnight. In the gut the same result is obtained in a matter of hours, operating first in the relatively acidic environment of the stomach, and then in the mild alkaline conditions of the duodenum, at 37 °C.
The released amino acids, together with di- and tri-peptides, are absorbed by enterocytes. The di- and tri-peptides are usually hydrolyzed into constituent amino acids within the enterocytes, and this explains why practically only free amino acids are present in the portal circulation.
References
- Bender D.A. Benders’ dictionary of nutrition and food technology. 8th Edition. Woodhead Publishing. Oxford, 2006
- Bender D.A. Introduction to nutrition and metabolism. 3rd Edition. Taylor & Francis, 2004
- Bhagavan N.V., Ha C-E. Essentials of medical biochemistry: with clinical cases. 4th Edition. Academic Press, 2011. doi:10.1016/C2009-0-00064-6
- Nelson D.L., Cox M.M. Lehninger. Principles of biochemistry. 6th Edition. W.H. Freeman and Company, 2012
- Rosenthal M.D., Glew R.H. Medical Biochemistry: human metabolism in health and disease. John Wiley & Sons, INC., Publication, 2009
- Stipanuk M.H., Caudill M.A. Biochemical, physiological, and molecular aspects of human nutrition. 3rd Edition. Elsevier health sciences, 2012